By William Pearce
In 1942, experience in the Pacific War made the United States Navy aware that their carrier-based fighter aircraft lacked the range needed to properly escort other aircraft while the home carrier stayed outside the combat radius of enemy aircraft. In addition, long-range ship-borne aircraft were needed to take the fight to the Japanese mainland. This prompted the Navy to search for a long-range, carrier-based fighter. The Boeing Airplane Company responded with its Model 400 design, which interested the Navy, and a contract for three prototypes was issued on 10 April 1943. The new aircraft was designated the Boeing XF8B.

The first XF8B (BuNo 57984) on a test flight along with a piggyback flight engineer hunched over behind the pilot. Note the two separate exhaust stacks in the cowling and the four stacks behind the cowling.
From the start, Boeing had sought an agreement with the Navy in which Boeing would be given almost full control of the project and would develop the aircraft as they thought best. Part of the reason for this request was that the buyer, whether Navy or Army Air Force, had a habit of constantly issuing minor changes which often resulted in some developmental chaos on the part of the aircraft manufacturer. The Navy had already issued specifications for the XF8B, which included a top speed of 342 mph (550 km/h), a minimum speed of 79 mph (127 km/h), a takeoff distance with a 25-knot (29 mph / 46 km/h) headwind of 262 ft (80 m), an initial climb rate of 3,760 fpm (19.1 m/s), and a ceiling of 30,000 ft (9,144 m). Since Boeing had agreed to these specifications, the Navy felt that Boeing’s interest to develop the aircraft with little interference was in good faith and begrudgingly consented to Boeing’s request.
The Boeing XF8B was an all-metal monoplane with a conventional layout. What was less conventional about the XF8B was its Pratt & Whitney R-4360 28-cylinder engine with contra-rotating propellers, its large size, the inclusion of an internal bomb bay, and Boeing’s intention for the aircraft to be more than just a long-range fighter but also to be used as an interceptor, level bomber, dive bomber, and torpedo bomber. The multi-role characteristic of the XF8B led Boeing to refer to it as the “Five-In-One” aircraft.
A mock-up of the XF8B was completed in September 1943 and was inspected by the Navy in early October. The design of the aircraft was finalized on 7 October 1943. The XF8B’s fuselage had flat sides with an arched top and a relatively flat bottom. It was formed by aluminum panels riveted to aluminum formers and longerons. The cockpit was above the wing’s trailing edge and enclosed in a rearward sliding bubble canopy. The control stick moved fore and aft like normal, but left and right movement was limited by a pivot point to the upper half of the stick. This design was adopted to save space in the cockpit. The pilot was protected by armored glass and an armor plate in front of the cockpit and an armored seat. A 40 US gal (33 Imp gal / 151 L) oil tank was positioned forward of the cockpit. Below the cockpit was a bomb bay that could accommodate up to 3,200 lb (1,451 kg) of ordnance. However, the bomb bay’s size limited what could be carried to four 250 or 500 lb (113 or 227 kg) bombs or two 1,000 or 1,600 lb (454 or 726 kg) bombs. One 2,000 lb (907 kg) bomb could also be accommodated. For long range flight, a 270 US gal (225 Imp gal / 1,022 L) fuel cell could be installed in the bomb bay. Two snap-opening doors enclosed the bomb bay and would automatically open and close during bomb release. The doors could open or close in under one second. At the rear of the fuselage was a fully-retractable tail wheel.

Taken before the aircraft’s first flight, this image shows the XF8B’s large flaps that extended back some 32 in (.813 mm) as they were deployed. The door in front of the cockpit was the air exit for the oil cooler. The hangar in the background was camouflaged to look like a building during World War II.
The aircraft’s wing was designed for +7gs and used a single spar. On the forward half of the wing, the aluminum skin was rivetted to the wing’s internal ribs and spot welded to the stringers. The rest of the wing used rivets. Each wing housed 192 US gal (160 Imp gal / 727 L) of fuel in three tanks: a 100 US gal (83 Imp gal / 379 L) tank between the fuselage and landing gear, a 42 US gal (35 Imp gal / 159 L) tank between the landing gear and gun bay, and a 50 US gal (42 Imp gal / 190 L) tank between the gun bay and wing fold. The main landing gear retracted to the rear with the tire rotating 90-degrees to lay flat in the wing. This gear retraction method was developed by Boeing in the early 1930s and licensed to Curtiss for the P-36 Hawk and P-40 Warhawk. When retracted, the gear strut was concealed by doors that joined a cover attached to the upper part of the wheel. The main gear had a track of 13 ft 4 in (4.06 m). The gun bay of each wing could accommodate three .50-cal machine guns with 400 rpg or three 20 mm cannons with 200 rpg. The armament could be mixed, but the 20 mm cannons would need to be outboard of the .50-cal machine guns because of clearance issues. The mounts used by Boeing to accommodate both the .50-cal machine guns and 20 mm cannons were designed by Vought for the XF5U Flying Flapjack. The outer 12 ft 4 in (3.76 m) of each wing folded up for storage on an aircraft carrier. Wing folding was controlled by electric motors and could only be accomplished after the wings were unlocked by one cockpit control, followed by engaging a covered switch. The wings would still not fold unless there was weight on the landing gear.
Large fowler flaps spanned the wing trailing edges from the fuselage to 20 in (508 mm) beyond the wing-fold point. The 20 in (508 mm) section of flap past the wing fold could be manually folded down 180-degrees. The flaps were powered by an electric motor and extended back approximately 32 in (.813 mm) and down to 35 degrees. The flaps would automatically retract when airspeed reached 150 mph (241 km/h) and automatically redeploy to the selected position when airspeed dropped to 120 mph (193 km/h). This was a safety feature to lessen the carrier pilot’s workload during a wave-off. The aileron occupied the rest of the wing’s trailing edge from the flap to the tip. The ailerons used booster tabs to lessen the input needed by the pilot. A hardpoint under each wing between the main gear and the fuselage could accommodate a bomb up to 1,600 lb (726 kg) or a 150 US gal (125 Imp gal / 568 L) drop tank. A hardpoint on the aircraft’s centerline would accommodate a torpedo and render the bomb bay inoperative, but it is not clear if such accommodations were ever made. In addition, later proposals included using the two wing hardpoints for torpedoes. There was also the capacity for eight rockets under the outer section of each wing.
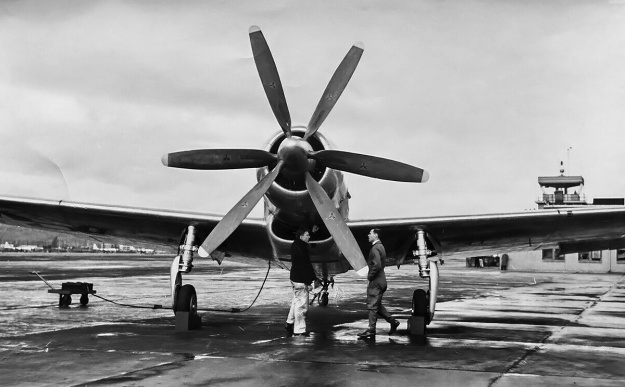
Test pilot Robert Lamson (right) and a crewman give scale to the very large size of the XF8B aircraft. Note that the machine guns were not initially installed in the first aircraft. The scoop under the cowling brought in air for the carburetor, intercooler, and oil coolers.
Like the rest of the aircraft, the XF8B’s tail was of all metal construction. The vertical stabilizer resembled that of the Boeing B-17 Flying Fortress and B-29 Superfortress. The rudder and elevators had balance tabs designed to maintain a constant force on the control surface. Under the tail was a tailhook that retracted flush with the belly of the aircraft.
At the front of the aircraft was the R-4360-10 engine enclosed in an elegant and fairly tight-fitting cowling. The R-4360-10 had a two-stage supercharger and produced 3,000 hp (2,237 kW) at 2,700 rpm. The first (auxiliary) stage was a large blower attached to the extreme rear of the engine and driven via a variable-speed fluid coupling. The second (main) stage had two speeds and was in the conventional supercharger housing between the carburetor and crankcase. The engine turned a six-blade, contra-rotating Aeroproducts propeller that was 13 ft 6 in (4.11 m) in diameter. This was basically the same engine and propeller combination used on the Republic XP-72, the difference being the XP-72 used a remote first (auxiliary) stage supercharger driven by a long shaft.
Engine cooling air was brought in the front of the cowling and expelled via cowl flaps around the upper part of the fuselage and slits for the exhaust stacks on the sides of the aircraft. The engine’s exhaust system was rather unusual and consisted of 14 exhaust stacks, with each stack serving two cylinders. On each side of the aircraft, four exhaust stacks were located in a slit behind the cowling. Another exhaust stack was forward of the slit and under the cowl flaps, and another stack protruded from the cowling forward of the cowl flaps. The last two stacks traveled through a passageway at the center of the scoop under the aircraft and expelled exhaust out of the bottom of the scoop.

The XF8B shortly after takeoff with a good view of the gear as it retracts. The doors close over the struts and meet the covers attached to the main wheels. Also just visible are the exhaust stacks in the bottom center of the scoop.
The scoop under the aircraft and just behind the cowling took in air for the carburetor, intercooler, and oil coolers via a complicated airbox. Induction air was fed from the airbox and into the auxiliary supercharger. After being pressurized, the air was fed back through an air-to-air intercooler housed in the airbox and then to the carburetor (and into the main supercharger). Air used to cool the intercooler was discharged via an exit flap forward of the bomb bay. An oil cooler was positioned on each side of the aircraft to the rear of the cowling. After passing through the airbox, cooling air was directed through the oil coolers and out exit doors on the upper sides of the fuselage, just forward of the cockpit.
The Boeing XF8B had a wingspan of 54 ft (16.46 m) and length of 43 ft 2 in (13.16 m). Its height was 12 ft 9 in (3.89 m) with the propellers down, 16 ft 3 in (4.95 m) with the propellers up, and 15 ft 10 in (4.83 m) with the wings folded. However, folding the wings required 20 ft (6.10 m) of vertical clearance. With the wings folded, the aircraft was 29 ft 4 in wide (8.94 m). The XF8B had a maximum speed of 432 mph (695 km/h) at 23,200 ft (7,071 m), 412 mph (663 km/h) at 14,500 ft (4,420 m), and 375 mph (604 km/h) at sea level. Takeoff distance with a 25-knot (29 mph / 46 km/h) headwind was 261 ft (80 m), and takeoff distance in still air with 35-degrees of flaps was 550 ft (168 m). The aircraft’s initial rate of climb was 3,110 fpm (15.8 m/s), and its service ceiling was 37,500 ft (11,430 m). The XF8B’s maximum range was 2,300 miles (3,701 km) at 225 mph (362 km/h) and 15,000 ft (4,572 m). This was with 384 US gal (320 Imp gal / 1,454 L) of fuel in the wing tanks, 270 US gal (225 Imp gal / 1,022 L) of fuel in the bomb bay, and 300 US gal (250 Imp gal / 1,136 L) of fuel in two drop tanks, for a total of 954 US gal (794 Imp gal / 3,611 L) of fuel. The aircraft had an empty weight of 14,100 lb (6,396 kg), a gross weight of 20,508 lb (9,302 kg), and a maximum weight of 22,960 lb (10,414 kg). Takeoff distance at 22,960 lb (10,414 kg) was 1,100 ft (335 m). It was believed that if the R-4360’s output were increased to 3,600 hp (2,685 kW), the XF8B could achieve 450 mph (724 km/h).
For a fighter comparison, the XF8B’s wingspan was 14 ft (4.27 m) more than a Republic P-47 Thunderbolt, and its empty weight was 2,000 lb (907 kg) more than the maximum gross weight of a North American P-51 Mustang. Overall, the XF8B was roughly the same size as a Grumman TBF/TBM Avenger but weighed 3,500 lb (1,588 kg) more.
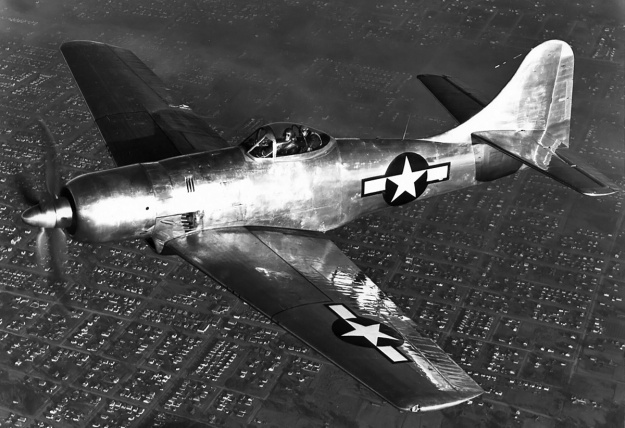
Lamson and probably Bud Zerega on a test flight in the first aircraft now fitted with machine guns. The aircraft stayed bare metal until mid-1946.
The XF8B program at Boeing was overseen by Wellwod E. Beall and Richard L. Stith, with Lyle A. Wood as the head engineer for most of the project. Although missing some components, a mock-up was inspected by the Navy in early October 1943. The Navy did not care for the bomb bay but was ultimately convinced by Boeing to keep it. The aircraft was built at Boeing Field in Seattle, Washington. Delays were experienced with the engine and propellers, but the first XF8B (BuNo 57984) was finished in late October 1944. The aircraft was left bare metal, and it was first run on 2 November 1944. Ground runs and taxi tests revealed a number of minor issues that were quickly fixed. The aircraft’s first flight occurred on 27 November 1944 and was piloted by Robert T. Lamson. The XF8B performed well during initial flight tests but aileron control was heavy.
The single-seat fighter was modified with a jump seat behind the pilot for a flight engineer. On 26 December 1944, Bud Zerega became the first XF8B “passenger,” carried aloft hunched over in the back of the cockpit. The second XF8B (BuNo 57985) was painted Navy Sea Blue and was completed on 31 January 1945. However, the aircraft was rolled off to the side to await delivery of its engine and propeller, which were very behind schedule. By this time, the Navy had begun to think the XF8B would be better suited as an attacker than a long-range fighter or any other role Boeing had envisioned.
On 13 February 1945, the first XF8B suffered a gear collapse during a high-speed taxi test. The aircraft was only lightly damaged, but the contra-rotating propellers were completely destroyed as the front blades were bent back into the rear blades. The cause of the accident was a faulty microswitch commanding the gear to retract. An anti-squat switch overrode that command because of the weight on the main gear, but as the taxi test was conducted, the wings generated lift and took weight off the main gear, enabling the faulty microswitch to retract the gear. The engine was replaced, and the aircraft was repaired in 19 days.

The second XF8B (BuNo 57985) with 150 US gal (125 Imp gal / 568 L) drop tanks delivered to the Army Air Force. This was the only aircraft with the tall canopy and blue spinner.
In March 1945, the XF8B was flown to the Naval Air Station at Patuxent River in Maryland. Here, the aircraft was evaluated by 31 pilots in 21 days. The overall impression was positive, although the aircraft’s brakes were noted as being weak and its ailerons heavy. The XF8B was then flown to Anacostia Naval Air Station near Washington, DC where another 10 pilots flew the aircraft over two days in early April. The first XF8B returned to Seattle on 9 April 1945. Flight testing continued until the aircraft was grounded in mid-August due to an issue with the engine’s gear reduction. While awaiting a new engine, the XF8B’s aileron control system was rebuilt to improve control, and the aircraft returned to the air on 22 October 1945.
After waiting nearly a year, the second XF8B (BuNo 57985) finally received its R-4360 engine and made its first flight on 27 November 1945, exactly one year after the first aircraft. By 26 December 1945, the aircraft had accumulated just over 11 hours when a stuck intake valve caused the engine to only produce idle power. Lamson was flying the aircraft at the time and decided to land at the nearest field, Everett Airport (not the current Paine Field). Everett Airport was also known as Ebey Island Airport and Snohomish County Airport, but it was often referred to as Marysville. It was a grass strip located on an island between Everett and Marysville. After the heavy XF8B touched down on the water-logged grass, the main gear sunk in, and the aircraft went up on its nose. The propellers were ruined again, but the rest of the aircraft had very little damaged. After three weeks of repairs, the second XF8B was flown back to Boeing Field.

XF8B BuNo 57984 now painted Navy Sea Blue with Lamson at the controls. Note the newly installed streamlined canopy. Reportedly, only the second aircraft (57985) was fitted with dive recovery flaps. However, there appear to be dive recovery flaps just aft of the gun ejection ports. The XF8B may have been the only aircraft to carry two different “Boeing” logos simultaneously (1930s Boeing airplane logo on the tail and 1940s Boeing script on the cowling).
On 13 February 1946, the second XF8B was flown to Wright Field, Ohio to be evaluated by the Army Air Force (AAF). The AAF had shown an interest in the XF8B, and the Navy agreed to sign the second aircraft over. A number of modifications were incorporated into BuNo 57985 at the direction of the AAF, including the addition of a dive recovery flap fitted outboard of the main gear wheel well. AAF evaluation continued until 3 April 1946, when an engine failure brought an end to the tests. The cause of the engine trouble was the failure of the second (main) stage supercharger drive.
The Third XF8B (BuNo 57986) made its first flight in March 1946. It did not incorporate the changes made to BuNo 57985 for the AAF, but it replaced the second aircraft for flying duties with the AAF. The aircraft was completed with a more streamlined canopy that was intended for all XF8B aircraft. In July 1946, BuNo 57986 was flown to Eglin Air Force Base in Florida to complete armament tests intended for BuNo 57985. The third XF8B was the only one to fire its guns in the air and drop bombs, even if they were just dummy bombs. Only .50-cal guns were tested, and they were fairly accurate. During one test, a ricochet broke the canopy, parts of which flew back and damaged the horizontal stabilizer. A gun blast tube failed in another test, resulting in that gun not being used for subsequent tests.
Problems with bombs included getting the bomb bay doors to open at higher speeds as well as the released bomb coming in contact with the quick doors as they closed. Also, the thin fins on bombs carried externally were prone to cracking due to the XF8B’s long flight duration and relatively high speed. The AAF tests concluded in December 1946, and they found the aircraft to be unsuitable as a dive bomber, due to longitudinal instability, and inferior as a fighter, due to its large size and slower maneuverability. At one point, the AAF had compared the XF8B to the XP-72, which seems like an unfair comparison given the different philosophies that went into the designs of the respective aircraft (multi-role vs all-out interceptor). The AAF felt that the XF8B was a satisfactory attacker and low-level bomber, but other aircraft adequately covered those roles.

The third XF8B (BuNo 57986) was completed with the streamlined canopy. Seen at Boing Field, the aircraft was delivered to the AAF to take the place of the second aircraft.
A new engine was installed in the first XF8B; a new streamlined canopy replaced the taller version, and it was painted Navy Sea Blue, like the two other aircraft. It was accepted by the Navy on 15 October 1946. The second aircraft was also fitted with a new engine, and it was accepted by the Navy on 5 November 1946. Following its time with the AAF, the third aircraft was accepted by the Navy on 1 August 1947.
The Navy’s opinion of the XF8B was similar to that of the AAF: the war was over and current aircraft were filling the various roles intended for the XF8B. On a crowded carrier deck, the large size of the XF8B meant that only half the number of aircraft could be accommodated compared to the Grumman F6F Hellcat and Vought F4U Corsair. While it was a good multirole aircraft, it did not particularly excel at any of the roles; it was a jack of all trades and a master of none. By some accounts, the Navy had reached out to Boeing in the spring of 1946 regarding a contract for 600 aircraft as torpedo bombers. The XF8B’s performance specifications combined with its ability to carry three torpedoes made the aircraft an attractive option. However, the managers at Boeing who fielded the Navy’s request were focused on B-29 production and turned it down.
In the end, the XF8B program cost twice the estimate, and the plane was 1,400 lb (635 kg) overweight. In addition, numerous delays were encountered that were sometimes the result of Boeing’s actions and sometimes the result of engine and propeller delivery issues. All three XF8Bs were eventually stored at the Naval Air Material Command in Philadelphia, Pennsylvania. BuNos 57984 and 57985 were struck off charge on 31 January 1948, and BuNo 57986 was struck off on 16 March 1950. Lamson attempted to purchase one of the XF8Bs, but the Navy refused, and all were scrapped.

XF8B BuNo 57986 sits in Philadelphia awaiting its day with the scrapper. The flap section that extended into the wing fold and the place it occupied in the folded wing can both be seen.
Sources:
Boeing XF8B-1 Five-In-One Fighter by Rick Koehnen (2005)
The Boeing XF8B-1 Fighter: Last of the Line by Jared A Zichek (2007)
U.S. Experimental & Prototype Aircraft Projects, Fighters 1939–1945 by Bill Norton (2008)
R-4360 Pratt & Whitney’s Major Miracle by Graham White (2006)